By Jordan McGlew1, Nick Jervis-Bardy1, Andrea Marsland-Smith2, Philip Mill2, Jonathan Ross1
1Orica Digital Solutions
2Alligator Energy
Introduction
In-situ recovery (ISR) is among the most sustainable and economically efficient techniques of mineral extraction and is widely used in uranium, copper, and potash mining. ISR involves the circulation of lixiviants through the subsurface mineralization using groundwater flow as the transport mechanism, with a subsequent hydrometallurgical processing plant at the surface.
The viability of ISR projects rely on a comprehensive understanding of the hydrogeological properties of the formation containing the desired mineralization as well as the necessary confining layers. Conventionally, this information is gathered from core samples and pumping tests which are both time-consuming and costly. The well-established technology of Nuclear Magnetic Resonance (NMR) extensively used in other industries, has recently been made available for the mining and groundwater industries, capable of resolving the complexity of hydrogeological systems.
The Borehole Magnetic Resonance (BMR) tool is a downhole geophysical instrument that interacts with the fluid contained in the pore space directly and (i) measures the amount of fluid (total porosity), (ii) differentiates between moveable and non-moveable fractions (effective porosity) and (iii) infers how easily the fluid is likely to flow through the porous medium (hydraulic conductivity) as a continuous log.
Using data from an investigation performed on an Australian uranium deposit as an example, we explore how the BMR can be used to successfully resolve the aquifer and aquitard boundaries and assist in the understanding of the aquifer properties itself.
In-situ Uranium Mining
ISR mining was developed in the 1970’s for extracting uranium from sandstone hosted deposits that could not be mined open cut or underground. Sandstone hosted deposits are one of the most common styles of uranium mineralization. These deposits form by the mobilization of uranium from source rocks by oxidized waters into aquifers, which then precipitates out in reduced conditions. A uranium ‘roll front’ forms as the oxidized waters carrying the uranium continue to flow into the aquifer (Figure 1).
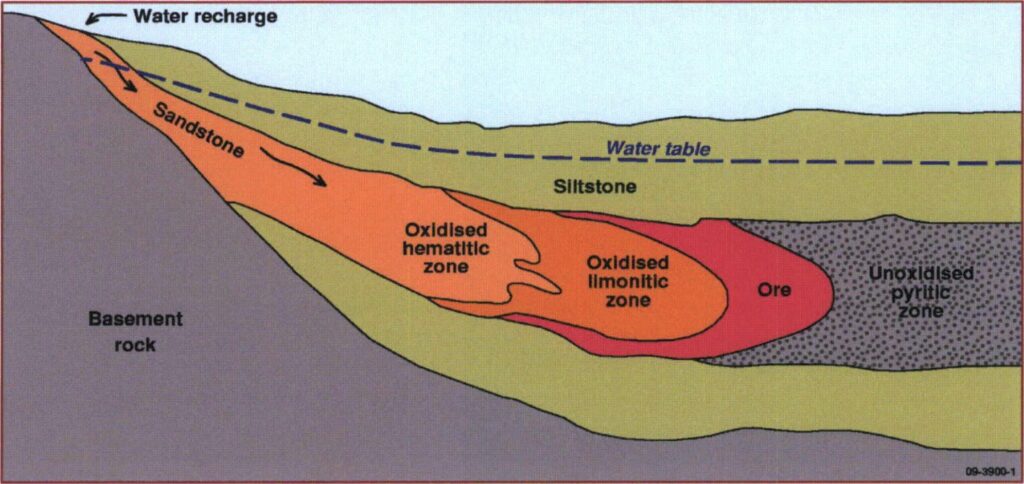
Figure 1. Diagrammatic cross-section of a roll front sandstone uranium deposit (Geoscience Australia, 2010).
ISR uranium mining accounts for 66% of the world’s uranium production (World Nuclear Association, 2022). It involves using formation water which has been conditioned to be either acidic or alkaline depending on the target uranium chemical make-up. The conditioned formation water is injected into the formation through injection wells. It then oxidizes and dissolves the uranium into the groundwater which is then brought to surface through extraction wells. The extraction wells are usually placed in the middle of a group of injection wells to keep the conditioned water from moving away from the wellfield (i.e., the mining zone). Once the uranium is mobile in solution, it is pumped to the surface via the well network to a plant for further processing. The uranium is collected via resin capture where the uranium binds to the resin. The barren solution is then reconditioned and recirculated back to the host formation and the cycle repeats until the uranium grade shows marked decline.
Understanding the porosity and more importantly, the permeability of the formation hosting the mineral deposit is critical in determining the feasibility of an in-situ project. There needs to be a moderate degree of permeability for the conditioned groundwater to flow through the formation with ease. The formations above and below (i.e., the confining layers to the mining zone) ideally need to be low permeability or not hydrologically connected to the mining zone.
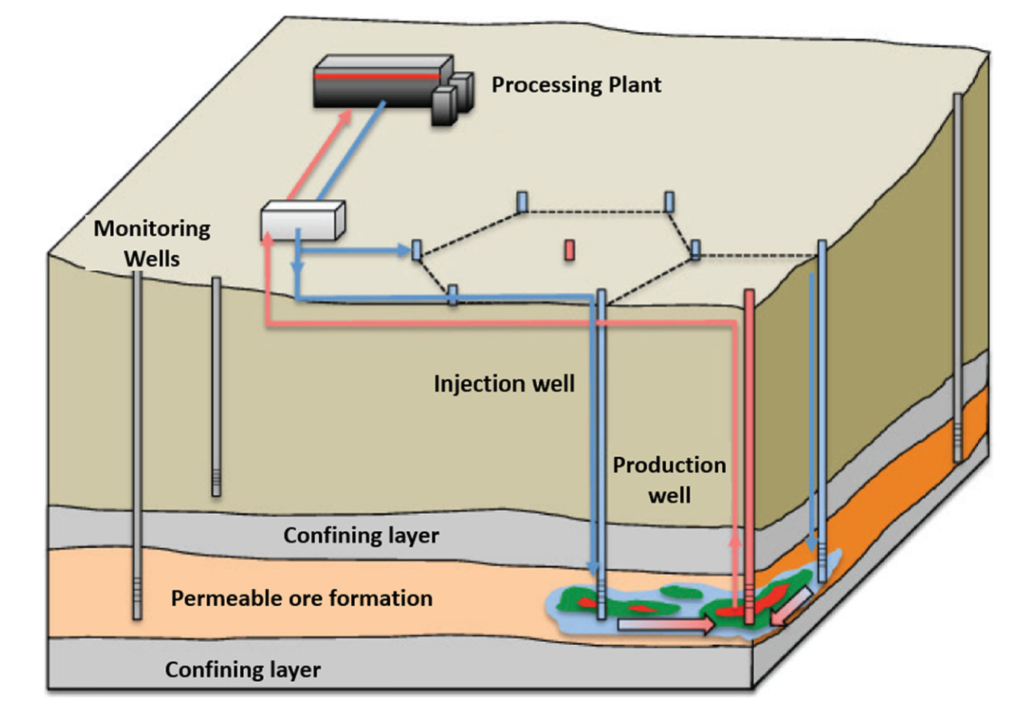
Figure 2. Schematic of uranium in-situ mining operations (Langanay et al, 2019).
Data Acquisition Problems for ISR Projects
Successful ISR exploration and mining is reliant on a comprehensive understanding of the hydrogeological context of the area of interest. Currently cores, pump tests and production data are used to assess the potential and the performance of a deposit.
Pore volume, being the total available pore space within the target deposit is a fundamental property used when assessing the quantity and makeup of the mining solution as well as predicting the length of wellfield recovery. Effective porosity and permeability are two parameters which are key for calculating the pore volume. Hydrogeological measurements are used to also characterize the host formation. Ideally this should be a permeable aquifer unit, bound by aquitards. This information is also useful for regulators to understand the effects of ISR on other aquifers.
At the early stages of assessing the ISR amenability of a deposit (i.e., exploration stage) pumping tests are not viable due to requirement of a well network in order to undertake such tests. In production, coring activities in active fields is difficult and when done, the process is slow from coring to receiving results as well as being expensive. Pumping tests require time, expertise, and excess water.
NMR Logging
Nuclear magnetic resonance (NMR) has been used in the resource industry since the 1960’s and can add valuable hydrogeological data to projects. The BMR tool uses the same principles as the MRI machines in the hospital, except instead of looking inwards, the BMR looks outwards. It measures the hydrogen response (or water response) within the saturated rock matrix. With this measurement, we can directly measure the total porosity of the rock as well as calculate a range of other variables, such as permeability and hydraulic conductivity.
The BMR has a permanent magnetic field which polarizes the hydrogen atoms to all align. Because they are all spinning in an upright position, it is difficult to get a detectable signal. The tool then sends out a radio frequency pulse which tips the atoms over in the excitation phase. Due to the tipping, the atoms are now resonating and emitting a detectable signal. The atoms begin to relax back to the upright position and then tipped again and again. The time is takes for the atoms to relax is known as the T2 distribution.
Case Study
South Australia currently has three (3) in-situ uranium projects, all at different stages. Heathgate Resources is currently producing from their Four Mile Mine through its Beverley Plant (https://heathgate.com.au/our-story/heathgate-story/), Boss Energy have the approval to restart production at the Honeymoon site (Boss Energy LTD ASX Release 6 October 2022) and Alligator Energy is working through approvals for a field recovery trial at Samphire (Alligator Energy ASX Release 25 November 2022).
The data in this case study is with thanks to Alligator Energy who recently announced they have found some of the highest uranium grades in the Kanaka sands at the Samphire site to date (Alligator Energy ASX Release 23 November 2022).
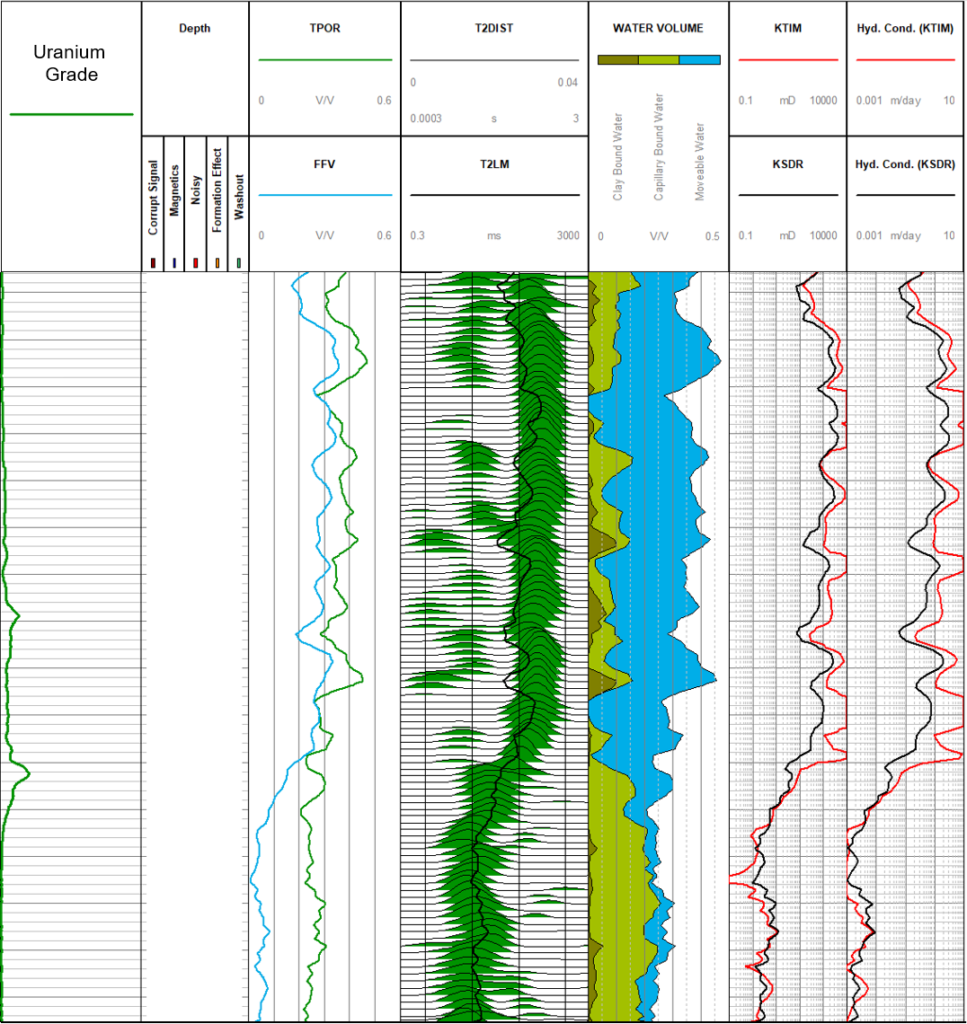
Figure 3. BMR log with uranium grade in track 1. Track 2 – Depth and flags. Track 3 – Total porosity (TPOR) and free fluid volume (FFV). Track 4 – T2 distribution and T2 log mean (T2LM). Track 5 – water volumes separated using T2 cut-off to separate clay-bound, capillary-bound and free fluid. Track 6 – Permeability calculated via the Timur-Coates (KTIM) method and the Schlumberger Doll Research (KSDR) method. Track 6 – Hydraulic conductivities calculated using the KTIM and KSDR permeabilities.
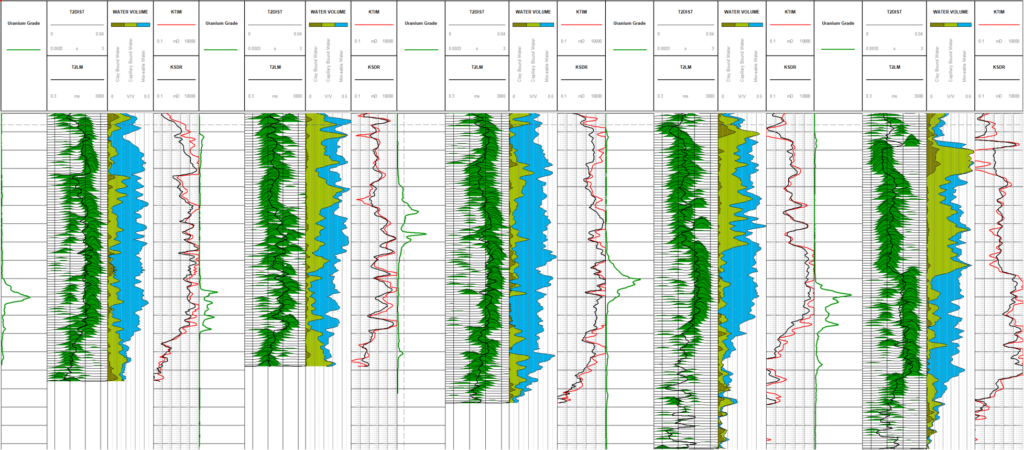
Figure 4. Cross section of results through the field. The BMR measurements indicate the uranium target is within the necessary highly permeable sands.
The targeted uranium is hosted in the Kanaka sands, an Eocene palaeochannel. The geology is a mix of fine-grained to coarse-grained sands representing the channel and floodplain depositional sequence with the target uranium located in the coarse-grained sands.
The BMR results show high porosity, high permeability, large amounts of moveable fluid and long T2 times (large pore spaces) in areas where the uranium grade is high suggesting the deposit is in the targeted palaeochannels and aquifer unit. Above and below this aquifer unit there is a decrease in permeability and the shorter T2 times indicate smaller pore spaces are present with the fluid bound in capillaries and clays. This suggests that the necessary aquitards are bounding the high uranium aquifer unit.
With permeability being a critical parameter in determining the feasibility of a uranium resource, the BMR measurements provided quantitative information about the permeability of the formation as well as how the water is sitting in the matrix, that is, bound or free.
Outcomes
Previously, the porosity and permeability measurements were qualitative. Reported by either geologists, which varies from person to person or from electrical logs which are influenced by the chlorides in the groundwater and require corrections. The BMR was able to produce direct total porosity measurements which are independent of the lithology – not affected by the lithology and quantitative permeability measurements. These permeability measurements gave confidence that the target was situated in permeable sands and were able to be used to prove this to the market.
The use of BMR also reduced the need for pumping tests at the current stage of the project’s development, allowing for a more cost-effective approach with the ability to narrow down where pumping tests would be more useful in the future.
In this case the BMR provided data which resulted in the confirmation that the deposit is ISR amenable. It allowed Alligator Energy to be able to quickly identify the host aquifer and confirm the presence of aquitards above and below the future mining zone. Generally, it was shown that the BMR is able to provide quick insights into aquifer and aquitard properties as well as give an indication of lithologies present by using the T2 distribution to characterize the pore-space distribution relating directly to lithology.
Acknowledgements
We would like to thank Alligator Energy for allowing me to present their data and for giving insights and support throughout. We would also like to thank the team at Orica Digital Solutions for their continual support and encouragement.
References
Alligator Energy, ASX Release 23 November 2022
Alligator Energy, ASX Release 25 November 2022
Boss Energy LTD, ASX Release 6 October 2022
Geoscience Australia (2010). Australia’s in situ recovery uranium mining best practice guide. Canberra, Australia.
Heathgate Resources, https://heathgate.com.au/our-story/heathgate-story/
Langanay, J., Romary, T., Freulon, X., Langlais, V., Petit, G., & Lagneau, V. (2021). Uncertainty quantification for uranium production in mining exploitation by In Situ Recovery. Computational Geosciences, 25(3), 831-850.
Authors Bio
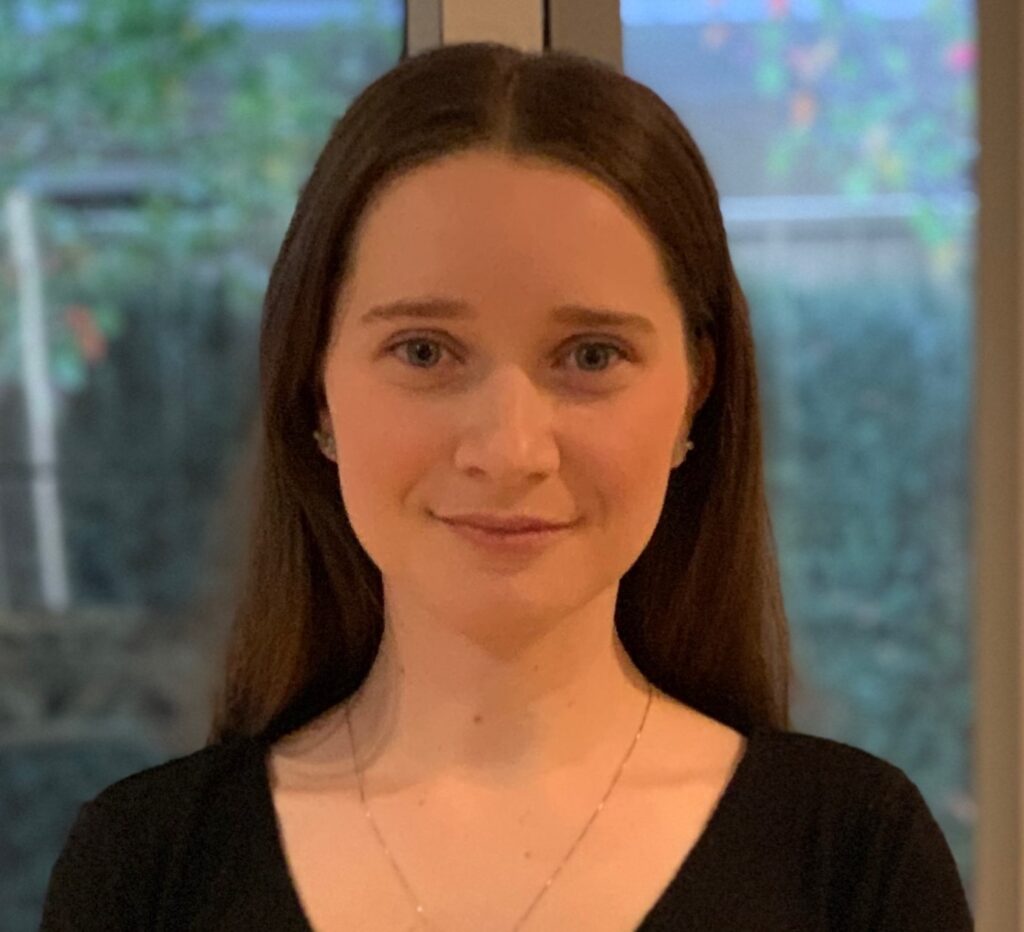
Jordan McGlew has 6 years’ experience working in the resource sector and is currently a Senior Geophysicist at Orica Digital Solutions in the Orebody Intelligence Division where she has been working with downhole geophysical data for the past 2 years. Her experience ranges from the conventional suite of data to the more advanced televiewer and NMR processing and interpretation. Jordan is currently studying a Master in Hydrogeology at UWA and has previously completed a Bachelor of Science in Environmental Biology and Applied Geology with First Class Honours from Curtin University.