By Luke Bowman1, Joshua Richardson2, John Gierke1, Fredy Cruz3, Rutilio Parada4
1Michigan Technological University, Department of Geological and Mining Engineering and Sciences
2Unaffiliated (at the time of data collection/processing, J. Richardson was affiliated with Michigan Technological University, Department of Geological and Mining Engineering and Sciences)
3Universidad de El Salvador – Facultad Multidisciplinaria Paracentral, Departamento de Ciencias Agronómicas
4Universidad Técnica Latinoamericana, Escuela de Ingeniería Agronómica
Abstract
Seismic refraction surveys were conducted on steep slopes of San Vicente Volcano, El Salvador, adjacent to the 2009 Hurricane Ida triggered failures and in areas that experienced surface cracks during Tropical Depression 12E in 2011. Surveys were conducted at the ends of the dry and rainy seasons in an attempt to discern changes in soil moisture and perched water tables. The survey design and execution were focused on high-resolution characterization to discern layering. The results were very precise and reproducible. Layering was evident in four of the six surveys collectively for the three sites. One site exhibited no apparent layering over the depth of study. The other sites showed layering but no apparent presence of a water table in either season, but a higher moisture content was observed at the end of the rainy season. Likely that the permeability of the deposits and the steepness of the slopes prevent a perched water table except for extreme rain events, which are associated with named storms. Shear-wave refraction surveys were also performed which yielded no additional insights into subsurface conditions but did provide direct measurements of shear wave velocities that were used to mathematically explore plausible scenarios for changing moisture and depth.
Introduction
Steep, volcanic slopes in tropical regions are highly susceptible to shallow, rainfall-induced landslides and debris flows/lahars (Bogaard et al., 2018; Capra et al., 2018; Frattini et al., 2004; Scott 2001; Scott et al., 2005). San Vicente volcano in central El Salvador received 355 mm of intense rainfall from Hurricane Ida rain over five hours, spanning 8-9 November 2009, which triggered shallow landslides on the northern flank and quickly transformed into lahars that inundated communities depicted in Figure 1. More than 200 people were killed (EM-DAT 2009), and the disaster ranks as the third most costly in El Salvador’s history with US$315,000,000 in damage (Tellman 2011; Bowman and Henquinet 2015; GOES 2009).
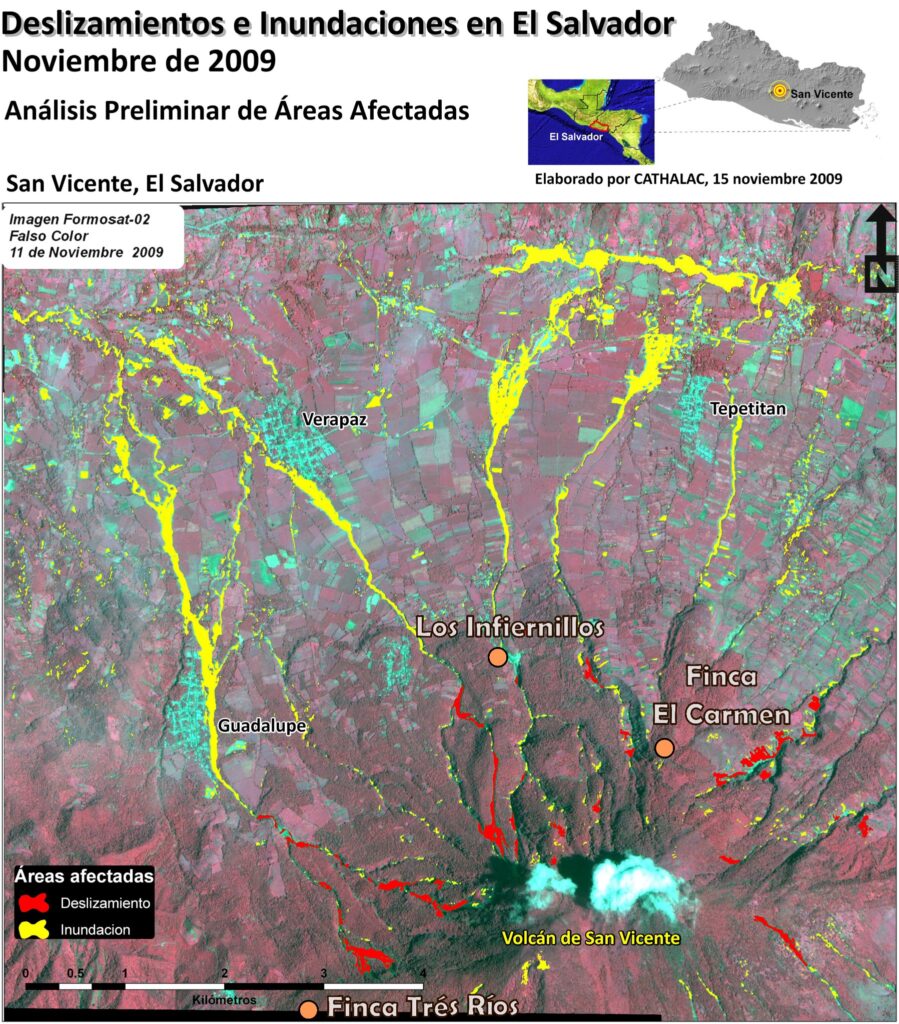
Figure 1. Landslides and debris flow inundation areas after Hurricane Ida, November 2009. (Modified from; SERVIR 2009). 13.595°N, 88.837°W, 2182-m above mean sea level. Orange circles indicate survey site locations (Finca Tres Ríos, Los Infiernillos, Finca El Carmen).
San Vicente Volcano has not erupted in the Holocene. Its thick soils are interbedded with Tierra Blanca ash layers from eruptions of nearby Ilopango Volcano. The ash layers have formed soils and been eroded and incised due to the tropical climate (Jibson and Crone 2001; Smithsonian-GVP 2013). The underlying old lava flows and hydrothermally altered, fine-grained clayey layers may slow vertical drainage from the surficial soils. Soil saturation may have reduced the strength of the slopes and caused the onset of the failures observed in 2009 (Rolo et al., 2004). Though landslides have occurred on both edifices, the older, more-weathered western edifice has historically experienced more landslide events. Even though five landslides were recorded over the last 350 years, no prior research was conducted to better understand rainfall-induced triggering mechanisms or educate at-risk populations to the hazards inherent to the area. Post-disaster inspection identified five major slope failures that caused supersaturated volcanic debris to flow into drainages and inundate communities on the northern flank of San Vicente Volcano (Schweig 2010).
Rainfall duration and intensity are widely known factors contributing to slope instability. A rainfall-intensity threshold for forecast-warning purposes does not exist for San Vicente Volcano. Seasonally perched aquifers forming above shallow, low-permeability fine-grained soil and/or rock layers have been identified as factors that trigger landslides (Terlien 1998; Van Asch et al., 1999). No hydrological nor stratigraphy data exist at San Vicente Volcano.
Rainfall amounts from Tropical Depression 12E in 2011 led to the appearance of surface cracks in some of the highest cultivated slopes on San Vicente (Bowman et al., 2016). These cracks caused significant concern for local officials and residents (Bowman, 2015). Whether the cracks were superficial and benign or expressions of impending failures like the 2009 catastrophe was impossible to conclude due to the lack of understanding of the slope conditions.
The goal of this research was to characterize hillslope lithology and ephemeral perched water layers in rugged, largely inaccessible, tropically weathered volcanic terrains. Smith et al. (2014) used Rocscience slope-stability software to evaluate the most probable slope failure triggers. Their fieldwork noted shallow bedrock was evident in some of the 2009 landslide scarps. A low-permeability bedrock layer serve as the foundation for a shallow, perched water table in the relatively permeable, overlying unconsolidated materials. Their modeling revealed that depth to the water table was a critical factor affecting slope stability. Perched water tables could reduce grain-grain stress on steep slopes and be a triggering mechanism leading to slope failure. Their work suggested that the uppermost layer of soil is largely composed of Tierra Blanca, and at very shallow depths, these soils were extremely permeable (Smith et al., 2014).
Near-surface geophysics can delineate geologic layering and the presence of saturated conditions (Crosta et al., 2005; Mezerreg et al., 2019; Abidin et al., 2012). Seismic refraction possesses a valuable combination of penetration depth and subsurface resolution. The modest equipment requirements make the technique amenable to surveying relatively steep and vegetated terrains. Selected areas of focus prioritized the characterization of the shallow conditions (depths to bedrock and water tables) near landslides from the November 2009 disaster and the vicinities where the 2011 (Tropical Depression 12E) surface cracks appeared. In addition to the novel context for this work (steep, volcanic slopes), innovative time-lapse seismic refraction surveys were performed to characterize seasonal water-saturation conditions to explore the potential for perched water tables on the steep slopes. This investigation does not claim to predict specific areas that might fail in the future, nor does it consider massive, sector collapse-type slope failures. A 2001 USGS study analyzing debris-flow hazards at San Vicente indicates that shallow landslides provoking small volume (100,000 – 300,000 m3) lahars are most likely, which occurred in 2009 (Major 2004).
Methods
Seismic refraction surveys were conducted at three locations in May and November 2013, which are months marking the transitional periods from dry-to-wet and wet-to-dry seasons, respectively (Protección Civil, 2011). Two sites were adjacent to two separate areas that failed in 2009, and a third site was proximal to surface cracks that appeared after heavy rainfall from Tropical Depression 12E in October 2011. The first set of surveys coincide with the very end of the dry season when soils would be at their driest state. The same three survey sites were revisited at the end of the rainy season. The three survey locations (Infiernillos, Finca El Carmen, Finca Tres Rios) were chosen based on accessibility and proximity to slopes known to have failed in the recent past (see Figure 1).
Infiernillos is on the eastern slope of a ridge that divides the twin edifices and is located west of hydrothermal vents. During heavy rainfall associated with Tropical Depression 12E, cracks formed at the crest of the ridge upslope from the survey site. Geothermal alteration of the surficial deposits may be more pronounced at this site because of the prevalence of hot springs. Slopes at higher elevations in this drainage failed in 2009, which triggered the most destructive lahar (Bowman 2015).
Finca Tres Rios is on the west edifice and was chosen due to its proximity to a series of landslides, many of which started after the January-February 2001 earthquakes and were reactivated during the rains from Hurricane Ida in 2009. The survey was conducted on a ridge with major slides on both sides further up-slope.
Finca El Carmen is on the northern slopes of the older, eastern edifice. Though this area did not experience large slope failures as the east edifice in 2009, at least seven surficial cracks were reported after 12 days of heavy rain from Tropical Depression 12E.
The seismic-refraction surveys were designed and conducted without a priori knowledge of the geology. Time-lapse seismic refraction was performed by reoccupying the three sites at the end of the rainy season and analyzing the waveforms to elicit differences, if any, in arrival times of the P waves, which would show seasonal changes in the shallow soil moisture or water table depths. A waveform comparison approach allowed detection of subtle changes in depth to potentially perched water tables and relative soil moisture distributions within the soil profile. Without relying solely on travel-time differences from subjective identification of waveform arrival (first-break picks), the actual waveforms were compared, improving the detection limits of the refraction method. To reduce ambiguity in differentiating changes in saturation from changes in lithology, both shear- and compressional-wave refraction surveys were performed.
A sledgehammer and metal plate were used to create the sound source (“shot”) for each survey. Each shot location was sampled with at least 20 hits and the results from each were “stacked.” The sledgehammer was equipped with an inertial switch with which to instantaneously trigger the timer in the seismograph. Waveforms were recorded using a 24-channel Geode Ultra-Light Exploration Seismograph connected to vertical geophones with a 4-Hz natural period. Sampling lengths were adjusted for each site in order to record all coherent arrivals. All waveforms were recorded at -0.2 s (before start time) with a 0.5-ms sampling interval. Waveforms were recorded prior to the start time in order to filter ambient noise from the data without introducing edge-effect artifacts. Geophones were placed at 2-m intervals from 14 to 60 m along each survey line to delineate the shallowest refractors for each of the survey sites. Figure 2 depicts a conceptual geologic model of the hillslopes and survey design. For the pre-rainy season surveys, redundant shot points were performed so that the stratigraphy could be determined, as the dry ground resulted in poor coupling between the steel plate and the ground. Some shot points were reoccupied in the post-rainy season survey so that time-lapse comparisons could be made and additional shot locations were occupied to clarify uncertainties in the stratigraphy identified in the May surveys. The first-arrival time picks were made using only the unfiltered data from the May surveys.
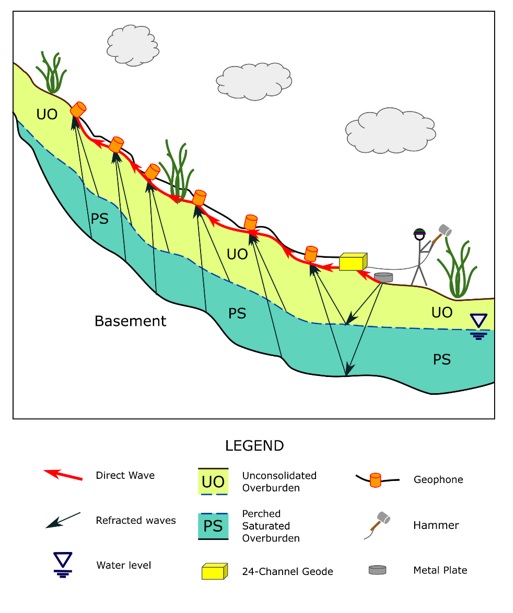
Figure 2. Conceptual geologic model of the three field sites and survey design.
Horizontally polarized shear (SH) wave refraction surveys were conducted using transversely oriented horizontal geophones. A buried, vertical plate was used as the shot source (transversely oriented). Each shot point experienced 40 hits, 20 in each direction. In this manner, through subtracting the positive transverse from the negative transverse shot directions, any P or vertically polarized shear (SV) waves would cancel, leaving only SH arrivals (Franklin 1979). Using the SH time-lapse could have aided in isolating the subsurface structure from changes in fluid saturation, and velocities from each survey could be used to estimate the shear modulus for fluid substitution. The surveys conducted to obtain SH wave refraction velocities were aimed at evaluating moisture content changes of the surface layer at Finca Tres Rios; however these data proved too noisy and are not included in this paper but are available from Bowman (2015).
Results and Discussion
The surveys revealed different soil and moisture profiles at each site. Two distinct layers (one refractor) were observed at Los Infiernillos, and three layers (two refractors) were interpreted at Finca Tres Rios. Finca El Carmen, east edifice where no large landslides occurred in 2009 but experienced surface cracks in 2011, showed no refractors. Because neither the wet- nor dry-season surveys at Finca El Carmen were able to detect a refractor (by our estimates, refractors deeper than 20 m could not be imaged), the results are not included herein but are published in Bowman (2015), which is publicly available online.
The upper layers at all sites are likely relatively loose, surficial deposits. Refractors appear as an underlying layer of highly fractured bedrock potentially mixed with soil, and a deeper wet and/or intact-rock layer.
Los Infiernillos is proximal to a 2009 lahar path and an active hydrothermal area. The survey line transects a crop field (downslope) and coffee plantation (upslope). The average slope is 26º. The survey point at 60 meters was the high point and designated as the origin with a survey elevation (zero) datum (Figure 3). Shot point locations occupied in May were conducted from the negative end of the survey line at 4, 6, 8, 10, and 12 m and from the positive end at 62, 63, 64, 66, 68, and 70 m. Many of these shots showed redundant results, and only two (shot points at 12 and 62 m) were required to make a complete interpretation. The first-arrival time “picks” are shown in Figure 3a for these two shot locations. Shots with further offsets (up to 12 m off both ends) did not show deeper refractors. The upper-layer velocities were determined to be 510 and 450 m/s from the positive and negative ends of the line, respectively. The up-dip apparent velocity of the refractor was determined to be 960 m/s and the down-dip apparent velocity was 900 m/s. The apparent dip of the refractor with respect to topography is only about 1º steeper than the slope (Figure 3b).
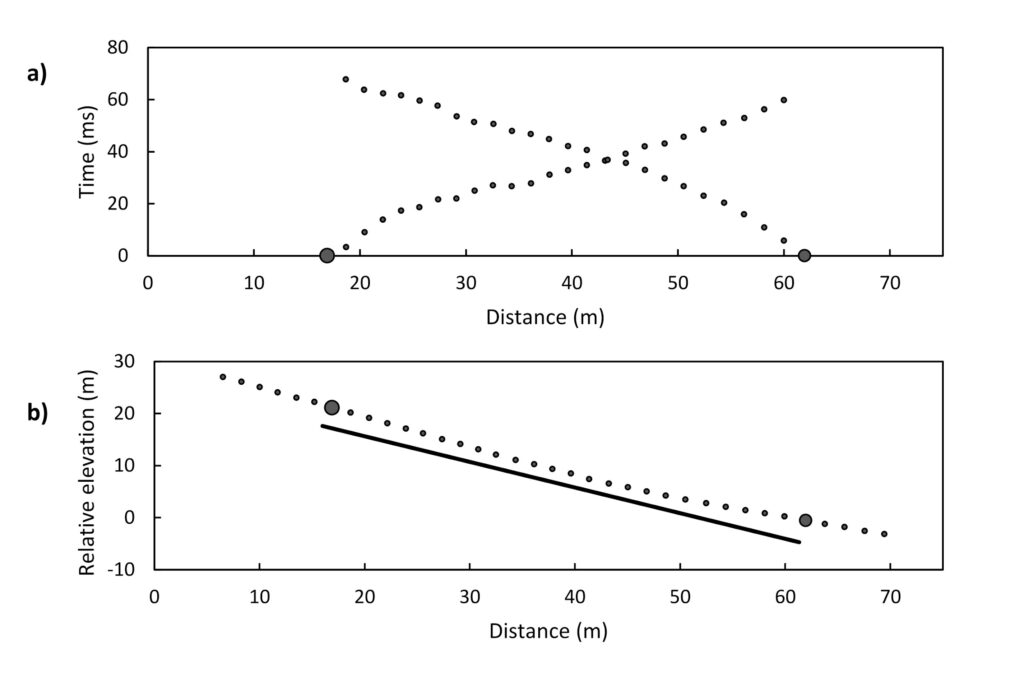
Figure 3. Los Infiernillos survey results from the dry season: (a) P-wave first break arrival times (small dots) from source locations at 62 and 12 m (large circles), and (b) interpreted refractor (solid line) from sources (large dots) at 12 and 62 m off-end shot points. Small dots are survey points along the line and x-axis is distance along the dark (interpreted) refractor line projected to horizontal. Note that both forward and reverse directions are required to accurately delineate a refractor not parallel to the survey surface topography.
The seismic refraction surveys at this site were repeated at the end of the wet season at shot locations of 8, 10, 62, 64, 66, 68, and 70 m. Four additional shot locations (2, 27, 43, and 60 m) were included to increase the confidence in the dry-season interpretation. To avoid damage to the corn crop grown during the rainy season, the geophone placement was shifted 2 m, so only 23 of the 24 geophones could be used for time-lapse comparison (see Figure 4). Nearly identical first-break picks from both ends of this survey were identified despite the shift, indicating an imperceptible change in layer properties ascertained from the seismic refraction methods. Figure 3 shows the time-lapse comparison from the shot point at 62 m. Neither the direct nor refracted arrivals show any significant time shifts.
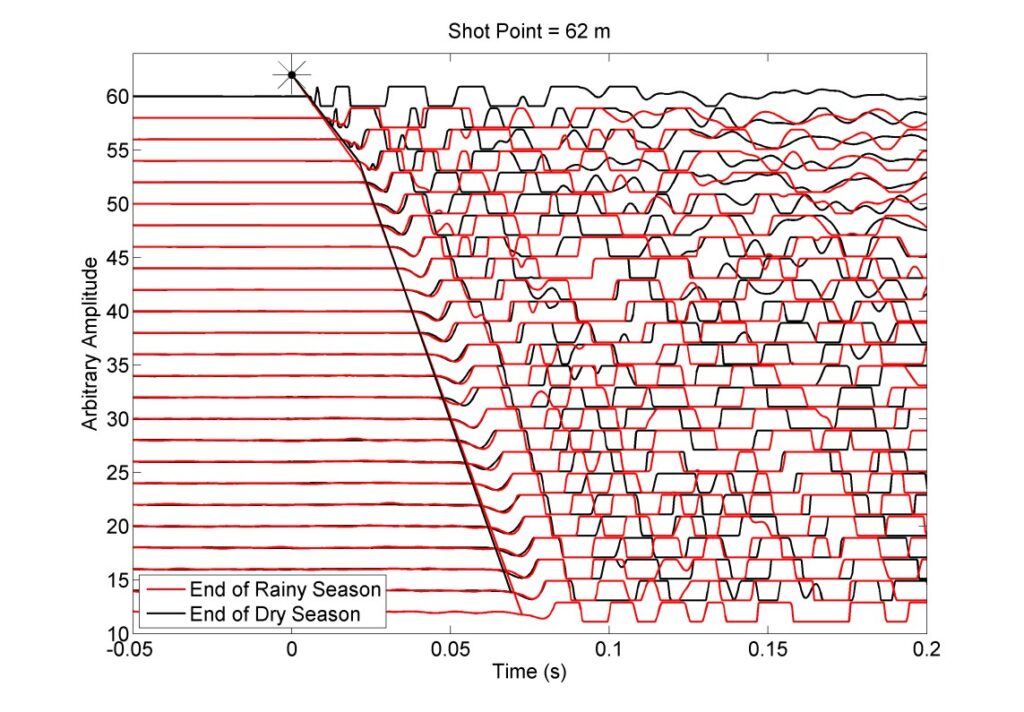
Figure 4. P-wave arrivals recorded from the shot point at 62 m individually normalized from each survey to demonstrate variations in first arrival waveforms from the end of the rainy season (red) to the end of the dry season (black). The survey location of each geophone is indicated on the y-axis.
Seismic data were acquired at Finca Tres Rios along a 27º slope. Shot locations occupied in May were conducted from the negative end of the survey line at 0, 2, 4, 6, 8, 10, and 12 m, from the positive end at 62, 64, 66, 68, and 70 m, and two split shots were performed at 28 and 48 m. Figure 5a shows the first-break picks selected at shot locations of 0, 12, 28, 48, 62, and 70 m. This survey revealed consistent direct arrivals at velocities of 410 and 420 m/s from the 12- and 62-m shot points, respectively. The data show a clear primary refractor from both ends of the survey and the split shots. A possible second refractor was imaged with data gathered at shot points taken far from the positive end of the survey line. A change in (relative) dip in the primary refractor is also evident around a position of 42 m. Figure 5b shows the simple interpretation of this site.
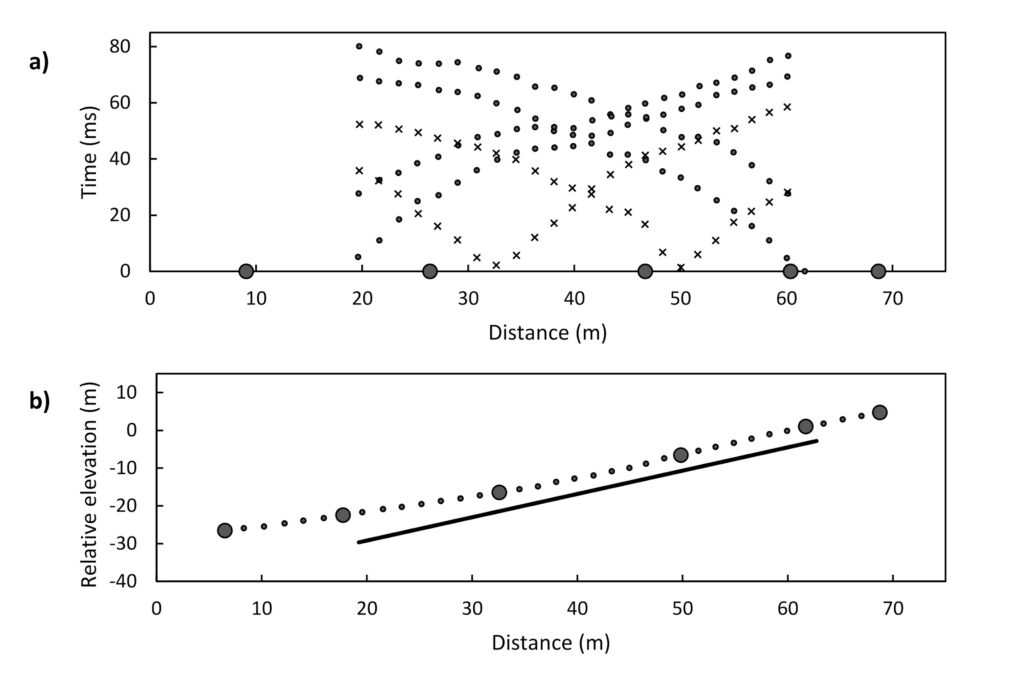
Figure 5. Finca Tres Rios survey results from the dry season: (a) P-wave first break arrival times (small dots) from source locations at 0, 12, 28, 48, 62, and 70 m (large dots), and (b) interpreted refractor (solid line) from sources (solid dots). Small dots are survey points along the survey line and x-axis is distance along the dark black (interpreted) refractor line projected to horizontal.
The Finca Tres Rios survey was repeated in November at shot points 0, 4, 12, 62, 66, and 70 m. Only the closest, on-end shot points at 12 and 62 m were used for the time-lapse interpretation, shown in Figure 6. First-break lines were drawn independently on each stage of the survey to highlight lithology changes. Both ends show distinctly different P-wave velocities between the May and November surveys for the direct waves through the upper layer, with the November survey displaying a lower velocity than the corresponding May survey. The two primary refractor slopes are essentially parallel, indicating that although the picks are shifted, the velocity of the refractor has not changed substantially. The shot point at 62 m also shows a slight difference in slope between surveys for the second refractor (third layer), but the signal-to-noise ratio deteriorated significantly for these offsets.
First-layer velocity in May were 10-14% slower than in November. This significant change in velocity is not attributed to survey uncertainty since this trend is apparent from both ends of the line. The refractor (second layer) shows less variation in velocity.
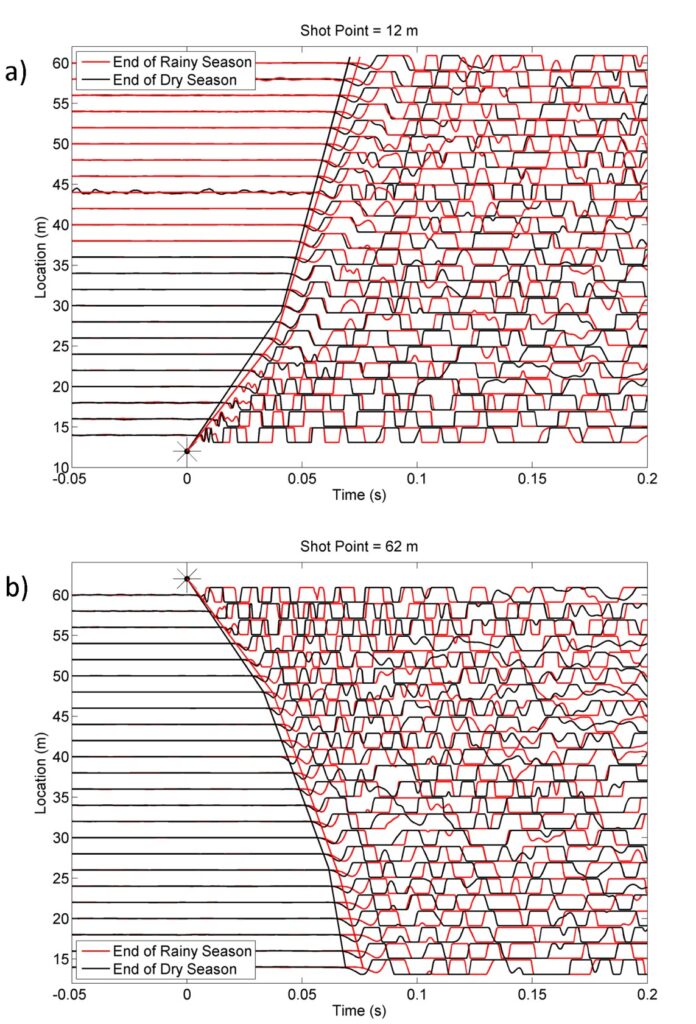
Figure 6. First breaks in both directions reveal decrease in wave velocity in November compared to May at the Finca Tres Rios site.
Saturation is almost certainly higher following the rainy season in November than it was at the end of the six-month dry season in May. Bowman (2015) details a series of calculations based on P-wave velocities from the surveys reported herein and estimates of shear moduli from S-wave surveys at the sites. The analysis yielded an estimate of the May water saturation at 32%, which is half of the water content determined from the November survey.
Synthesis
Saturation differences due to accumulated rainfall between the dry and wet seasons might help understand whether water table depths varied seasonally and thus might contribute to shallow landslides toward the end of the rainy season and during intense tropical rainfall events. Detecting perched water tables was not possible—not necessarily due to the methods used but because of other potential factors. This fieldwork was conducted after two consecutive comparatively light rainfall years. One explanation is that saturated layers could not be imaged because not enough precipitation had accumulated throughout the rainy season to fully saturate soils above impermeable layers.
Not only was it a relatively dry year, the tail end of the rainy season was noticeably drier than average years. Even though the rainfall was well below average, it is sufficient to result in an increase in the water table height of a few meters, unless the infiltrated rainfall escaped downslope without significant accumulation. Significant increases were not observed and the water table is deeper or the shallow volcanic deposits are permeable enough to allow the infiltration to flow downslope without significant buildup.
Conclusions
Time-lapse shear- and compressional-wave seismic refraction methods showed high repeatability and were able to image distinct stratigraphic layers. Perched water tables either were absent or not discernible. From the apparent decrease in shallow layer velocity from the dry to the rainy season, we can conclude that although the saturation of the shallow layer logically increases throughout the rainy season, the permeable texture of the soils likely prevented this layer from becoming saturated. The approaches tested herein show promise that for normal wet-season conditions, a broader selection of survey sites, and/or a campaign combined with other geophysical methods, future campaign results might indicate the existence of perched aquifers or other specific conditions lending to unstable slopes in volcanic terrains.
Acknowledgments
The research and activities outlined in his paper were supported financially by the National Science Foundation (NSF) Partnership for International Research and Education (PIRE) Grant 0530109 and National Academies of Sciences (NAS) Partnerships for Enhanced Engagement in Research (PEER) Cycle 1 – Project 354. The authors are also grateful for the assistance in the field provided by colleagues: Jorge Alas, Santiago Crespin, Adonay Hernandez, Melecio Ayala, and many more field assistants!
References
Abidin, M.H.Z., Saad, R., Ahmad, F., Wijeyesekera, D.C. and Baharuddin, M.F.T., 2012, Seismic refraction investigation on near surface landslides at the Kundasang area in Sabah, Malaysia. Procedia Engineering, 50, pp.516-531.
Bogaard, T., & Greco, R., 2018, Invited perspectives: Hydrological perspectives on precipitation intensity-duration thresholds for landslide initiation: proposing hydro-meteorological thresholds. Natural Hazards and Earth System Sciences, 18(1), 31-39.
Bowman, L. J., 2015, The 2009 and 2011 hazard events at San Vicente Volcano, El Salvador: Vulnerability, resettlement, and disaster risk reduction (Doctoral dissertation, Michigan Technological University).
Bowman, L. J., & Henquinet, K. B., 2015, Disaster risk reduction and resettlement efforts at San Vicente (Chichontepec) Volcano, El Salvador: toward understanding social and geophysical vulnerability. Journal of Applied Volcanology, 4(1), 1-18.
Bowman, L. J., Gierke, J. S., & Centeno, J. F. C., 2016, University contributions to risk reduction following a disaster: A case study of reorienting natural hazards research efforts at San Vicente volcano, El Salvador. Geological Society of America Special Papers, 520, 335-346.
Capra, L., Coviello, V., Borselli, L., Márquez-Ramírez, V. H., & Arámbula-Mendoza, R., 2018, Hydrological control of large hurricane-induced lahars: evidence from rainfall-runoff modeling, seismic and video monitoring. Natural Hazards and Earth System Sciences, 18(3), 781-794.
Crosta, G.B., Imposimato, S., Roddeman, D., Chiesa, S. and Moia, F., 2005, Small fast-moving flow-like landslides in volcanic deposits: the 2001 Las Colinas Landslide (El Salvador). Engineering geology, 79(3-4), pp.185-214.
EM-DAT, 2009, The International Disaster Database. Retrieved 3/12, 2014, from http://www.emdat.be/search-details-disaster-list.
Frattini, P., Crosta, G. B., Fusi, N., & Dal Negro, P., 2004, Shallow landslides in pyroclastic soils: a distributed modelling approach for hazard assessment. Engineering Geology, 73(3–4), 277-295.
GOES, 2009, El Salvador: Damage, Loss, and Needs Assessment for Disaster Recovery and Reconstruction after the low pressure system associated with Tropical Storm Ida. GFDDR, Global Facility for Disaster Reduction and Recovery.
Jibson, R. W. and A. J. Crone, 2001, Observations and recommendations regarding landslide hazards related to the January 13, 2001 M-7.6 El Salvador earthquake, US Department of the Interior, US Geological Survey.
Major, J. J., Schilling, S.P., Pullinger, C.R., Escobar, D.C., 2004, Debris-flow hazards at San Salvador, San Vicente, and San Miguel volcanoes, El Salvador. Geological Society of America Special Papers 375.
Mezerreg, N.E.H., Kessasra, F., Bouftouha, Y., Bouabdallah, H., Bollot, N., Baghdad, A. and Bougdal, R., 2019, Integrated geotechnical and geophysical investigations in a landslide site at Jijel, Algeria. Journal of African Earth Sciences, 160, p.103633.
Protección Civil, 2011, Plan Invernal. San Vicente: 44.
Rolo, R., Bommer, J. J., Houghton, B. F., Vallance, J. W., Berdousis, P., Mavrommati, C., & Murphy, W., 2004, Geologic and engineering characterization of Tierra Blanca pyroclastic ash deposits. Geological Society of America Special Papers, 375, 55-68.
Schweig, E. S., Major, J.J., Godt, J.W., 2010, Reconnaissance Survey of Landslide Hazards at Volcán de San Salvador and Volcán de San Vicente, El Salvador, and evaluation of Servicio Nacional de Estudios Territoriales (SNET) operational capacity and capability. USGS, USGS: 9.
Scott, K. M., Vallance, J. W., Kerle, N., Luis Macías, J., Strauch, W., & Devoli, G., 2005, Catastrophic precipitation-triggered lahar at Casita volcano, Nicaragua: occurrence, bulking and transformation. Earth Surface Processes and Landforms, 30(1), 59-79. doi: 10.1002/esp.1127
Scott, K. M., 2001, Catastrophic debris flows transformed from landslides in volcanic terrains: mobility, hazard assessment, and mitigation strategies, U.S. Dept. of the Interior, U.S. Geological Survey.
SERVIR, 2009, Deslizamientos e Inundaciones en El Salvador Noviembre de 2009: Analisis Preliminar de Areas Afectadas Retrieved 12 August, 2011, from http://www.servir.net/images/desastres/2009-11-11_inund_SV/sv_guadalupe_nov09_med.jpg
Smith, D. M., Oommen, T., Bowman, L. J., Gierke, J. S., & Vitton, S. J., 2015, Hazard assessment of rainfall-induced landslides: a case study of San Vicente volcano in central El Salvador. Natural Hazards, 75(3), 2291-2310.
Smithsonian-GVP, 2013, Volcano Info – San Vicente. Global Volcanism Program. Retrieved March 8, 2014, from http://www.volcano.si.edu/volcano.cfm?vn=343070.
Tellman, E., 2011, Community Resilience and Hurricane Ida: How Marginalized Salvadorans Lacking NGO and Governmental Support Cope with Climate Shock. Climate Change and Migration: Rethinking Policies for Adaptation and Disaster Risk Reduction. M. S. Leighton, X.; Warner, K. Bonn, Germany, United Nations University. 15/2011: 14.
Terlien, M. T. J., 1998, The determination of statistical and deterministic hydrological landslide-triggering thresholds. Environmental Geology 35(2-3): 124-130.
Van Asch, T. W., J. Buma, et al., 1999, A view on some hydrological triggering systems in landslides. Geomorphology 30(1): 25-32.
Author Bios
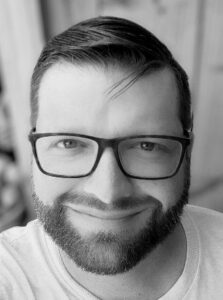
Luke J. Bowman, Ph.D. is a research assistant professor of geology at Michigan Technological University. He holds B.A., M.S., and Ph.D. degrees in Geology. He has lived in Honduras as a Peace Corps volunteer, in El Salvador as a Peace Corps Response volunteer and Fulbright Student Scholar, and in Ecuador as an assistant professor at Yachay Tech University. His research examines social dimensions of natural hazards that affect at-risk communities. [email protected]
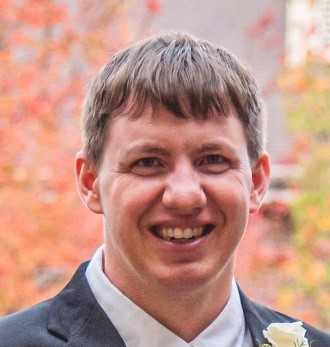
Joshua P. Richardson, Ph.D., is an applied geophysicist currently employed in the use of seismic technology to produce oil and gas from the offshore deepwater Gulf of Mexico, United States. He holds B.S., M.S., and Ph.D. degrees in geophysics from Michigan Technological University. While currently employed in the energy industry, his background includes volcanic hazard seismology and glacial seismology. [email protected]
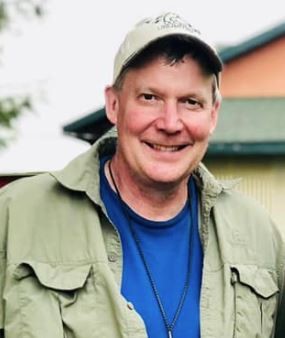
John S. Gierke, Ph.D., P.E., is a professor of geological engineering at Michigan Technological University, a career in research and education spanning more than 3 decades. He holds B.S. and M.S. degrees in Civil Engineering and a Ph.D. in Environmental Engineering. He and his students have worked on groundwater exploration and characterization projects using field geophysics and remote-sensing methods in the Keweenaw Peninsula of Michigan and in volcanic terrains in Nicaragua, Ecuador, and El Salvador. [email protected]
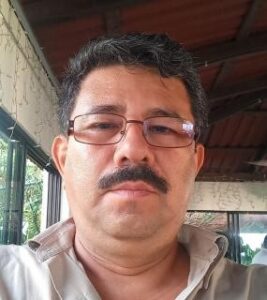
Jose Fredy Cruz is an Agricultural Engineer and is a professor in the Department of Agronomic Sciences of the University of El Salvador. He specializes in the areas of soil and water conservation. He has coordinated different training processes in Disaster Risk Management. He has a M.S. Degree in Training for Development and Educational Change. He has worked on soil and water quality analysis in small-scale organic farms. [email protected]
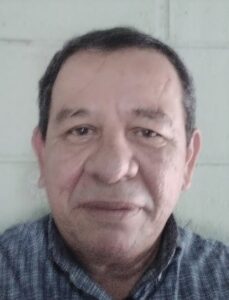
Rutilio Antonio Parada Galán is an Agricultural Engineer, has diplomas in Environmental Management for the Management of Hydrographic Basins, Hydrogeology, and in Food and Nutritional Safety. He has postgraduate degrees in Community Forestry at FLACSO Guatemala, and in Risk Management with Gender Equity. He has 30 years of work in the areas of risk management, GIS, and environmental management and territorial management in El Salvador and Guatemala. He currently serves as the Director of the School of Agricultural Engineering at the Latin American Technical University. [email protected]